Solar Radiation: A closer look at the Sun as a source of Energy
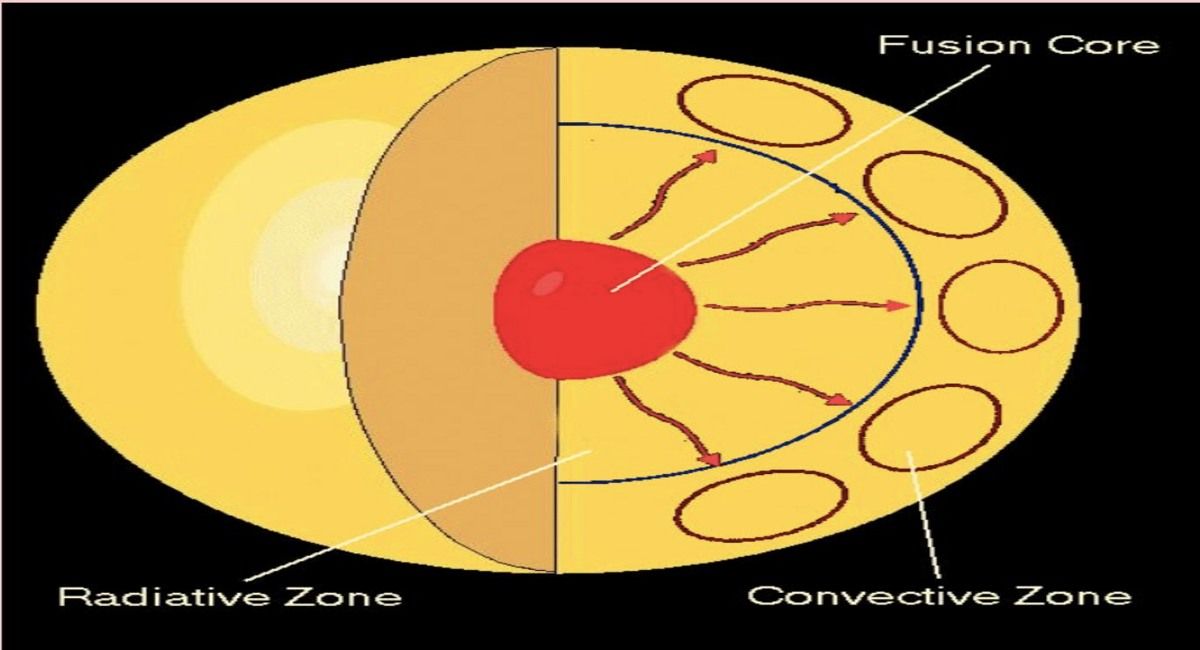
Energy is found all around us and has different sources. One of the main sources of energy on Earth is the sun. In fact, it can be said that the energy from the sun is the original source of most of the energy found on Earth. How? It is well-known that life on Earth is directly dependant on the sun. All living beings require the sun, either to store food, grow food or just to survive. The sun heats the Earth’s surface, causing the air above the surface to heat up too. This creates wind currents that can be used to power wind turbines. The sun also heats the water bodies, evaporating the water in them to form clouds. These clouds condense and cause rainfall, making rivers and streams to flow. These give way to hydropower. And lastly, the energy from the sun can be used directly in solar thermal energy and photovoltaics. Therefore, most forms of Energy depend on either the heat or light of the sun. But where does the Sun get this energy from and how does it reach the Earth? This article sheds light at the sun’s energy, how it reaches the Earth, and how it is quantified for optimal uses.
Radiation is a form of energy transfer that happens through space or through the medium of a material. This energy is transmitted or emitted in the form of waves or particles. All objects that have a temperature above absolute zero i.e. zero Kelvin (-273°C) emit energy. This energy is in the form of electromagnetic radiation. What this means is that the energy is composed of electric and magnetic fields which vary simultaneously while radiating. This is seen in the image below, where the blue wave can be considered as the electric field and the red wave can be considered as the magnetic field:
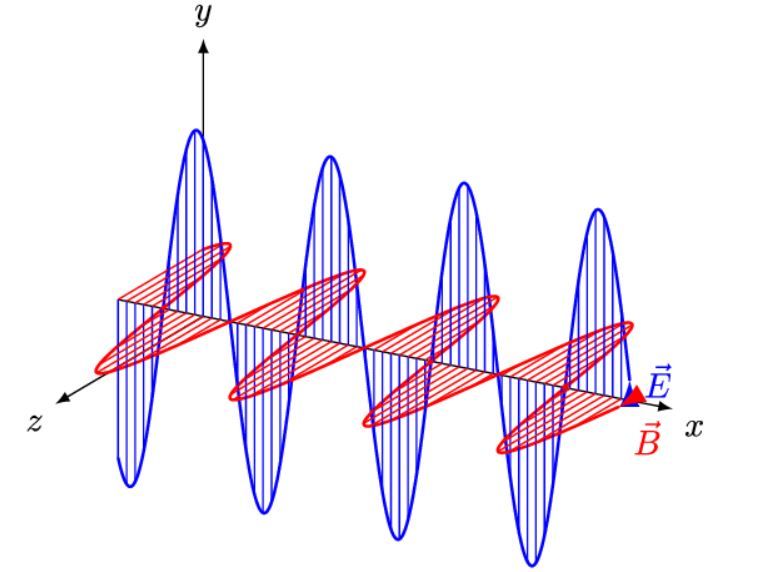
Various common sources of light, such as the sun and incandescent bulbs, can be classified as blackbody emitters. A blackbody absorbs all the radiation that is incident on its surface and emits radiation based on its temperature. Blackbodies get their name because when they emit radiation which is not in the visible spectrum, they appear to be completely black. This is because all the wavelengths have been absorbed. Blackbody sources which are compliable to photovoltaic applications emit light in the visible range. For instance, the sun’s light is in the visible range.
The sun is more than just a source of light. It is a spherical celestial object that is made up of gas. Its core consists of hydrogen atoms that undergo nuclear fusion to create helium atoms. These reactions cause the temperature of the core of the sun to be over twenty million Kelvin. The radiation from the core gets absorbed by a layer of Hydrogen atoms which are closer to the surface of the sun. Hence, the inner radiation is not visible. However, the heat transfer takes place through this layer of hydrogen atoms by convection. The different layers of the sun are depicted in the following image:
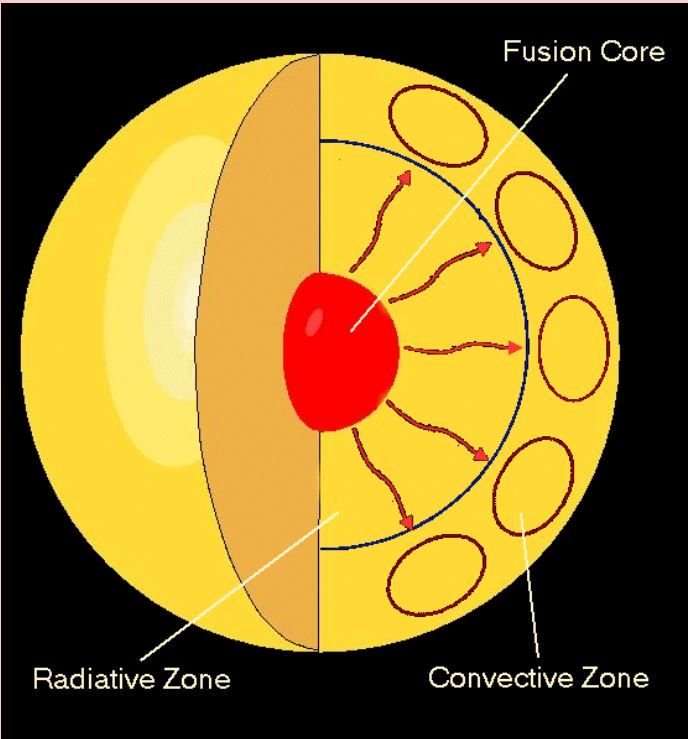
The sun’s surface is called the photosphere. Its temperature is around 6000 Kelvin. However, there are different approximations of the photosphere’s temperature for different scenarios. The total power emitted by the sun is 3.9 x 1026 watts which is determined by multiplying the sun’s surface area with its emitted power density. Light from the sun appears yellow or white as it is composed of multiple lights of different wavelengths. When the sun’s light passes through a prism or water droplets, the light scatters into a band of multiple wavelengths. This explains the phenomenon of rainbows. Even after scattering, some wavelengths are still invisible to the eye and thus, cannot be seen in the scattered band of light.
Even though the power the sun emits is massive, only a fraction of this power has an effect on objects in space at some distance from the sun. This is because the total power of the sun is spread out across the surface area of the object. The measure of this is solar irradiance. This is the power density incident on an object because of the sun’s illumination. The solar irradiance on an object at some distance from the sun is found by dividing the total power emitted from the sun by the surface area over which the sunlight falls. The spreading out of the power density causes the solar irradiance to decrease with the object’s distance from the sun increasing.
The sun’s radiation is relatively constant on the earth’s atmosphere. However, different reasons cause the radiation to vary on the earth’s surface. These reasons include atmospheric effects (including absorption and scattering), local variations in the atmosphere (such as water vapour, clouds, and pollution), latitude of the location, and the season of the year and the time of day. The effect these conditions have include variations in the overall power received, the spectral content of the light and the angle from which light is incident on a surface. Also, the variability of the solar radiation at a particular location increases dramatically. This difference can be caused by local effects such as cloudy skies as well as other effects such as the length of the day at particular latitudes. Therefore, cloudy skies do not affect deserts while the seasonal changes are not as significant in equatorial regions. These atmospheric conditions also affect photovoltaic applications as we will now see.
The main effects atmospheric conditions have on photovoltaic applications include a reduction in the power of the solar radiation due to absorption, scattering and reflection in the atmosphere. There is also a change in the spectral content of the solar radiation due to some wavelengths absorbing or scattering more. A diffuse or indirect component is also introduced in the solar radiation. Finally, the local variations in the atmosphere have additional effects on the incident power, directionality and spectrum. As the sun’s radiation passes through the atmosphere, the photons are absorbed by the gasses, dust and aerosols. Some gases such as ozone, carbon dioxide, and water vapour have very high absorption of photons. This absorption results in deep troughs in the spectral radiation curve. Specifically speaking, much of the far infrared light that is over 2 µm is absorbed by water vapour and carbon dioxide while most of the ultraviolet light below 0.3 µm is absorbed by ozone. This absorption has a relatively minor impact on the overall power received by the sun. The main factor that reduces the power is the air molecules and dust that absorb and scatter light. The power reduction is dependent on the length of the path the light has to cross in the atmosphere. So when the sun is overhead, the light appears white whereas, during the rise and set of the sun, the path is long. This means that the light has higher energy meaning that it is absorbed and scattered more effectively. This is why during sunrise and sunset, the shade of the sun is redder. Scattering is another effect the atmosphere has on sunlight. One of the mechanisms is called Rayleigh scattering. This is caused by molecules in the atmosphere. Rayleigh scattering is effective particularly on light that has a short wavelength (blue light). In addition to Rayleigh, another phenomenon is Mie scattering. This is caused by the aerosols and dust particles which scatter the light.
An important ratio that is considered in photovoltaic applications is the Air Mass. Air Mass is the length of the path that sunlight takes take through the atmosphere, normal to the shortest length it takes (when the sun is overhead). Air Mass quantifies the power of light reduced when the sun’s light crosses through the atmosphere and gets absorbed by particles the air. The following image better explains the concept:
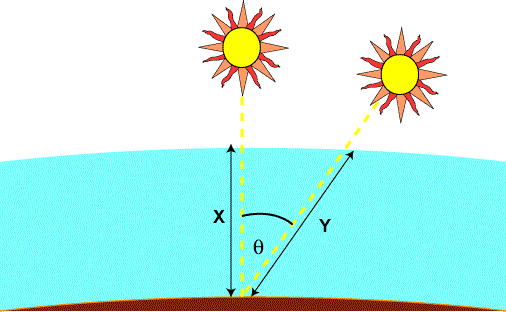
The angle theta in the above image can be used to determine the air mass as follows:
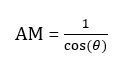
Since the cosine of zero is 1, that is why when the sun is right above us, the Air Mass is 1. The efficiency of PV applications depends on the spectrum and power of the incident light. Therefore, there are standardised power and spectrum defined for radiation both outside the earth’s atmosphere and at the earth’s surface. The spectrum at the earth’s surface is AM1.5G or AM1.5D. Here, G stands for Global (includes both direct and diffuse radiation) and D stands for Direct (only includes direct radiation). The global spectrum is 10% higher than the direct spectrum. These yield 970 W/m2. But since there are variations, this number is approximated to 1 kW/m2. Outside the Earth’s atmosphere the standard spectrum is called AM0 as the light does not pass through the atmosphere at any stage. This spectrum is specifically used to predict the expected performance of cells in space.
The sun was the only way to determine what time it is in medieval times. Until late 1800s, time was determined using the Local Solar Time (LST). In LST, noon is when the sun is right above the Earth. Local time (LT), which is the time we see on our clocks and watches, usually varies from LST. This is because of the oddness of the Earth’s orbit. Human adjustments such as time zones and daylight saving also make LT and LST differ.
The sun’s movement causes the changes in days and nights. It also determines the intensity of light on earth. When the sun’s rays are perpendicular to the absorbing surface, the power density on the surface is equal to the incident power density. However, as the angle between the sun and the absorbing surface changes, the intensity on the surface is reduced. The angle between the sun and a particular location on Earth depends on the longitude of the location, the time of year and the time of day. Also, the time at which the sun rises and sets depends on the longitude of the location. There are different angles that are associated with the sun’s radiation being incident on the earth. These are declination angle, elevation angle and Azimuth angle. The declination angle is the angle between the equator and another imaginary line drawn from the centre of the Earth to the centre of the sun. It is denoted by ‘d’. Declination varies with the season. This is because of the tilt of the Earth on its axis of rotation and the rotation of the Earth around the sun. The declination would always be 0° if the Earth was not tilted on its axis of rotation. However, the Earth is tilted by 23.45°. Thus, the declination angle varies plus or minus 23.45°. Only at the spring and fall equinoxes is the declination angle equal to 0°. The following image shows the declination angle:
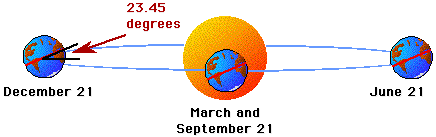
The elevation angle is the measure of the angular height of the sun in the sky measured from the horizontal. Altitude and elevation are both also used to describe the height above sea level. At sunrise the elevation is 0° and when the sun is directly overhead it is 90°. The elevation angle varies throughout the day. It also depends on the latitude of a particular location and the day of the year. The following image shows the elevation angle:
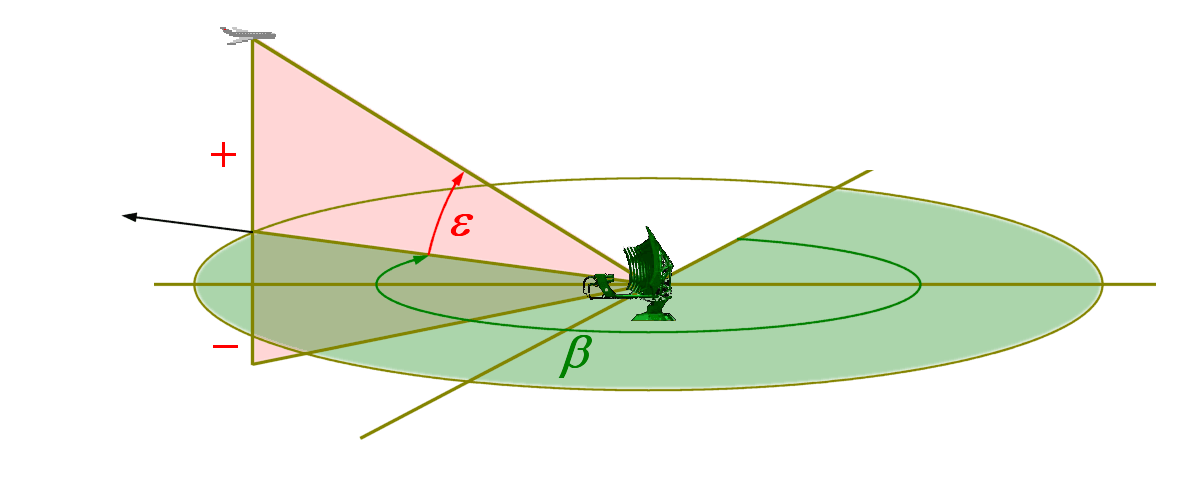
The Azimuth angle is the direction from which the sunlight is coming as if the earth was a compass. The azimuth angle varies throughout the day. At the equinoxes, regardless of the latitudes, the sun rises directly east and sets directly west. This makes the azimuth angles 90° at sunrise and 270° at sunset. In general however, the azimuth angle varies with the latitude and time of year. The following image shows the azimuth angle:
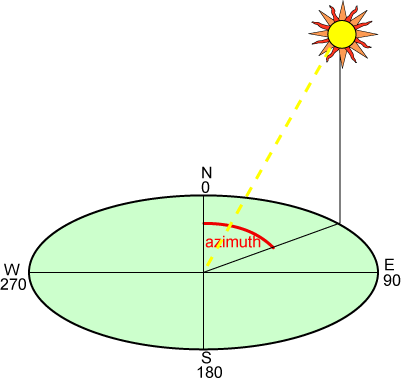
The various quantities associated with the sun can be used to analyse and interpret solar radiation data. This data helps in knowing how much sun will be available in a region of the earth on a certain day. The equation of the sun’s position in the sky throughout the year can help determine the maximum amount of solar insolation on a surface at a particular tilt angle. This can be calculated as a function of latitude and day of the year. Solar radiation of a region can be determined in various ways. These include:
- Typical mean year data for a particular location
- Average daily, monthly or yearly solar insolation for a given location
- Global isoflux contours either for a full year, a quarter year or a particular month
- Sunshine hours data
- Solar Insolation Based on Satellite Cloud-Cover Data
- Calculations of Solar Radiation
In order to accurately measure solar radiation, a pyrometer is placed at a location for a few years. This is a very cumbersome process, however, as this is meant to be done for at least a decade or more where the direct radiation is measured every few minutes. Instead, averages are taken of data over weeks, months and years to present a more straightforward representation. The local solar data is described most commonly through Typical Meteorological Year data (TMY). In order to determine TMY data, different meteorological measurements are taken at hourly intervals over a few years to create a picture of the local climate. An average of the yearly data neglects the amount of variability. Hence, the month that is most typical of the location is selected. For each month, the average radiation over the whole measurement period is determined. This is done along with the average radiation in each month during the measurement period. The data for the month that has the average radiation most closely equal to the monthly average over the whole measurement period is then chosen as the TMY data for that month. This is repeated for all the months in the year. At the end, the months are added together to give hourly samples for a full year. The total amount of radiation received by a PV module, G, is composed of direct (beam), B, and diffuse, D, components. TMY data consists of both the diffuse and direct components’ data. And this is how TMY data is used to determine solar radiation data for PV applications.
We have looked at a glance at what radiation and blackbody radiation are. We have also seen how the sun generates energy and uses electromagnetic radiation to transmit energy in the form of heat and light to the Earth and other celestial bodies. We looked at the effect solar radiation has on itself in Earth’s atmosphere. The different atmospheric conditions were discussed that affect solar radiation. We developed an understanding of how the energy from the sun is quantified in ratios and angles which function as the performance parameters of PV applications. Finally, we looked at how solar radiation data is collected, analysed and interpreted for better prediction. This further helps in determining how much sunlight would be available in a region at a particular time. Hence, the concepts of and related to solar radiation and the data they provide are essential to solar design engineering.