Solar Microgrids: Designing and Implementing Off-Grid Solutions for Resilient Energy Supply
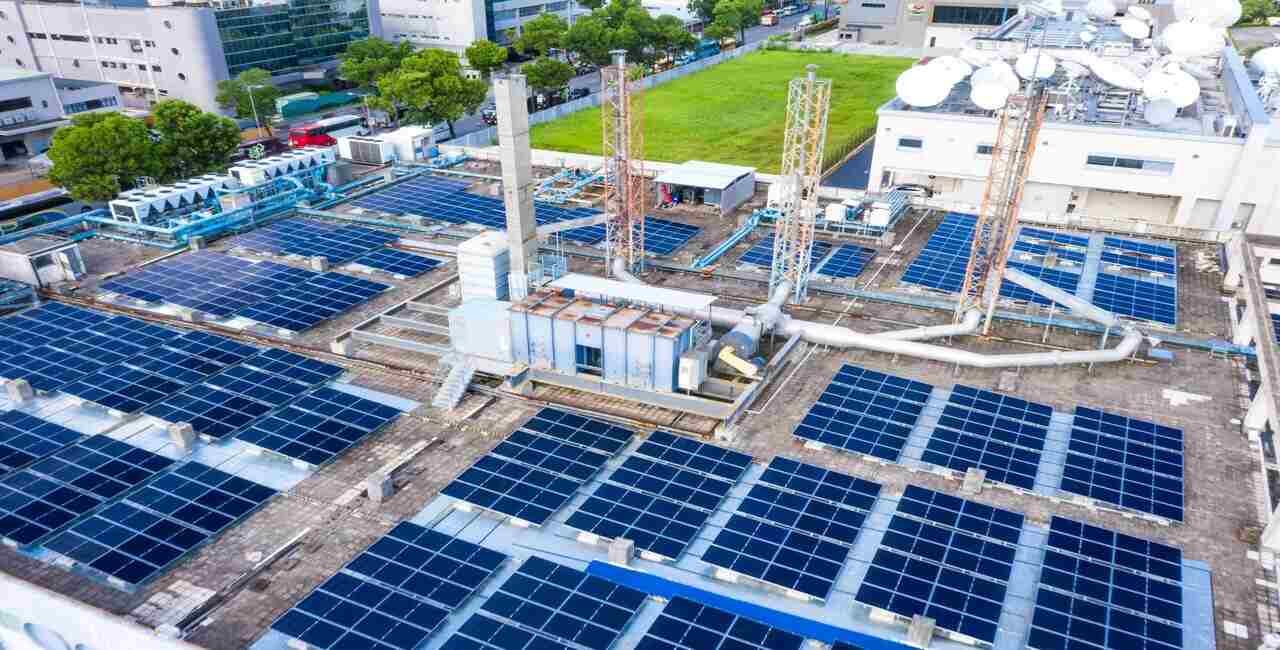
The search for sustainable and resilient energy solutions has accelerated in an era marked by rising energy consumption and worries over climate change. Decentralized energy systems have come to be seen as a potential alternative to existing centralized power grids, which are finding it difficult to keep up with the rising demand for electricity and their vulnerability to natural catastrophes.
Solar microgrids are one of them, and they have drawn a lot of interest because of their potential to completely alter the energy landscape by providing localized, off-grid solutions that boost energy resilience, encourage environmental sustainability, and stimulate economic growth.
This article discusses the complexities of solar microgrid design, the success stories of their actual implementation, and obstacles that must be removed for them to reach their full potential. We can pave the way for an energy supply that is not just dependable but also resilient and sustainable by adopting solar microgrids.
Introduction to Solar Microgrids
With a growing focus on sustainable and renewable energy sources, the global energy landscape has seen a substantial transition recently. The idea of solar microgrids, which combines solar power generation with localized energy delivery, is one potential breakthrough in this field. These microgrids are altering the way we produce and use power, and they have a wide range of uses, especially in rural places.
Understanding the concept and benefits of solar microgrids
An energy system that combines solar photovoltaic (PV) panels, energy storage options (such as batteries), and intelligent control systems is known as a solar microgrid. Depending on the particular requirements of the community or region they serve, these microgrids can run independently or be linked to the main grid.
The main objective of solar microgrids is to improve energy security, resilience, and sustainability, while also reducing greenhouse gas emissions and dependence on fossil fuels.
The benefits of solar microgrids are manifold:
- Resilience and reliability: By making the grid less vulnerable to outages and disturbances, solar microgrids improve the resiliency of the energy supply. Even during grid failures, they can keep supplying electricity to vital buildings like hospitals, shelters, and communication hubs.
- Energy independence: Solar microgrids enable communities to generate their energy, decreasing their dependency on centralized power generation and fossil fuels. This is crucial in regions with poor access to dependable grid infrastructure.
- Reduced environmental impact: By generating power from clean and renewable sources and reducing the negative effects of conventional energy production, solar microgrids help to lower greenhouse gas emissions and air pollution.
- Economic benefits: Because solar microgrids use cheap and plentiful solar energy, they can eventually result in cost savings. They also generate prospects for local employment in the fields of installation, maintenance, and operation.
- Scalability and flexibility: Solar microgrids may be created to supply all the energy requirements of a small town, a neighborhood, or even a single building. As energy demands increase, their modular architecture makes straightforward expansion possible.
Exploring Applications of Off-Grid Energy Supply in Remote Areas
Providing off-grid energy supplies to isolated and underserved communities is one of the most attractive uses for solar microgrids. Access to dependable energy is still a problem in many parts of the world, especially in remote and rural areas. To close this energy gap, solar microgrids are a practical option:
- Rural electrification: Rural electricity is made possible by solar microgrids in places where expanding the main grid is not viable financially. By introducing vital amenities like lights, communication, and necessary services that improve quality of life, they empower communities.
- Healthcare and education: In rural locations, solar-powered microgrids can provide a constant supply of electricity to hospitals and classrooms. This improves the delivery of healthcare, permits the use of medical technology, and increases children's educational opportunities.
- Agricultural production: Reliable energy from solar microgrids enables irrigation systems, cold storage, and agro-processing, enhancing agricultural production and raising farmers' incomes in the area.
- Economic development: By enabling small enterprises to work longer hours and make use of contemporary technologies, access to electricity boosts regional economies.
In conclusion, solar microgrids are a revolutionary development in the field of renewable energy. They are an effective instrument for solving energy poverty in rural regions, boosting sustainability, and advancing local development since they can deliver dependable, clean, and decentralized electricity.
Solar microgrids can empower local communities all around the world and contribute to a more sustainable energy future as technology develops.
Solar Microgrid Components and Configuration
To offer a dependable and resilient power supply, particularly in distant or off-grid locations, a solar microgrid is a decentralized energy system that combines solar power generation with energy storage and cutting-edge control technologies. A solar microgrid system's essential components include solar panels, batteries, inverters, and controllers. Together, these parts efficiently capture, store, transform, and manage solar energy.
Critical Components of a Solar Microgrid System: solar panels, batteries, inverters, and controllers
Solar Panels
The foundation of every solar microgrid is a solar panel, sometimes referred to as a photovoltaic (PV) panel. The photovoltaic effect is used by these panels to convert solar energy into electrical energy. They are made up of several semiconductor-based solar cells that, when exposed to light, produce direct current (DC) power. The efficiency of the solar cells, the angle and orientation of the panels, and the amount of sunlight received all affect the solar panels' overall power production.
Batteries
By storing extra energy produced during the day for use at night or during times of low solar irradiance, batteries serve a significant function in a solar microgrid. The microgrid's capacity to produce uninterrupted electricity is improved by battery storage, which also lessens dependency on backup generators and the main grid. Depending on parameters like energy density, cycle life, and maintenance needs, several types of batteries, such as lithium-ion, lead-acid, or flow batteries, can be utilized.
Inverters
Inverters are in charge of transforming the direct current (DC) power produced by the solar panels into alternating current (AC), which is the typical form of electricity utilized in most homes and businesses. Inverters control the flow of power between the solar panels, batteries, and the load in addition to these other functions. Some cutting-edge inverters also include grid-forming capabilities, which let the microgrid run independently from the main grid.
Controllers
Intelligent systems that govern the functioning of different microgrid components are known as controllers. They guarantee that the solar panels, batteries, inverters, and loads are properly coordinated. To fulfill demand and maintain the microgrid's stability and dependability, controllers optimize the energy flow. Additionally, they permit functions like load shedding during times of high demand or limited resources.
Designing the Optimal Configuration for Specific Energy Needs and Environmental Conditions
The optimal solar microgrid layout depends on balancing energy needs with environmental considerations. Examining load patterns, peak demand, and key loads in great detail is necessary to configure the system to meet particular energy requirements. This helps determine the size of solar panels and battery storage, guaranteeing a steady flow of electricity.
At the same time, it is crucial to comprehend the local environmental factors, such as sun irradiation, shade, and weather fluctuation. The best energy is captured when panels are positioned and oriented properly.
The battery's capacity is designed to retain more energy during solar-rich times, reducing power shortages during cloudy or nighttime hours. Inverter choice is influenced by load type, capacity, and the microgrid's ability to function independently. Energy distribution, load priority, and potential grid interactions are all governed by a solid control approach. Integrating redundancy raises dependability even more.
The optimum arrangement is responsive to the environment and coordinates energy production, storage, and distribution with the energy demand profile. Solar microgrids can effortlessly meet energy demands while adjusting to the dynamic interaction between energy usage and the environment thanks to their all-encompassing architecture.
Grid Integration and Energy Storage
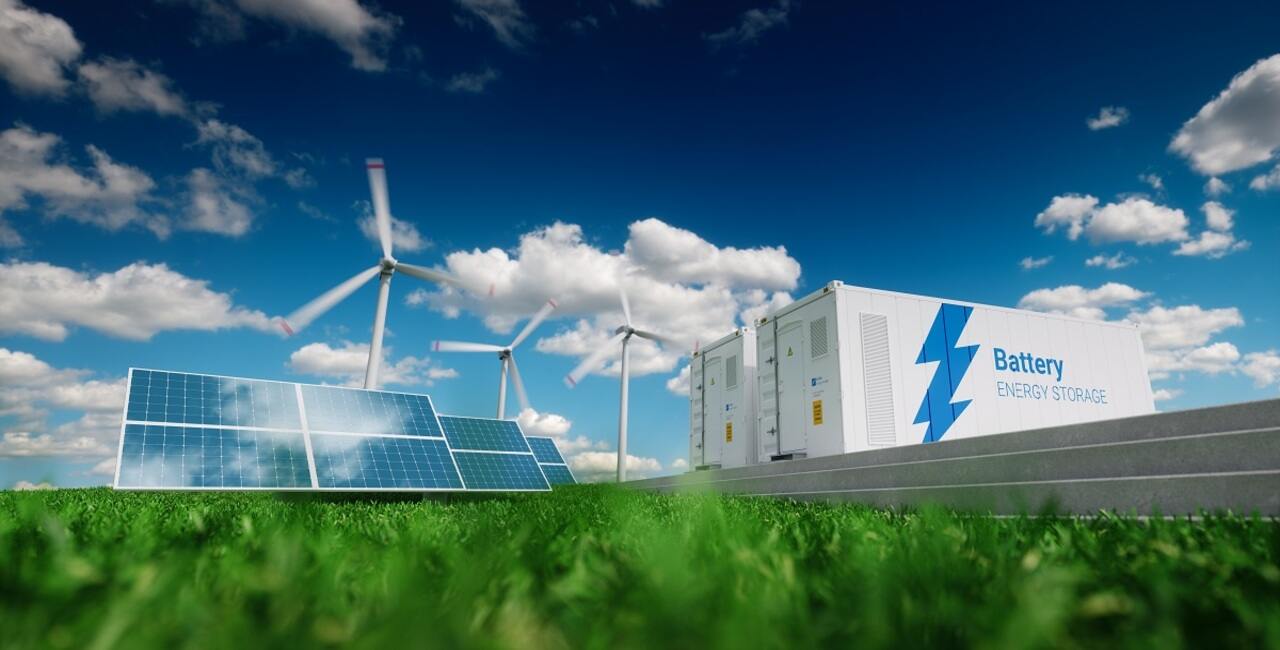
Integrating renewable energy sources and utilizing cutting-edge energy storage technologies are crucial steps in the direction of a stable and sustainable energy future. To maximize energy use, improve grid stability, and foster a resilient energy supply, multiple energy sources, load centers, and storage systems must be seamlessly interconnected. The notions of grid-tied and islanded microgrid topologies, where energy storage emerges as a key to stability, are particularly clear examples of this complicated equilibrium.
Examining Grid-tied and Islanded Microgrid Configurations
Energy distribution and resilience are exemplified through grid-tied and island microgrid systems, respectively. A grid-tied microgrid allows electricity to flow in both directions and smoothly integrates distributed energy resources (DERs) into the primary utility system. In addition to encouraging sustainability, this structure makes it easier to contribute excess energy to the grid during periods of peak generation.
An islanded microgrid, on the other hand, runs independently and is not linked to the main grid, assuring energy supply reliability in off-the-grid locations or during grid outages. It can independently support essential infrastructure thanks to localized generation and storage. Energy storage, a key component in both systems, balances supply, and demand, stabilizes fluctuations, and facilitates black start capabilities.
Finally, whereas islanded microgrids support energy security and self-sufficiency, grid-tied microgrids improve overall grid efficiency and include renewable energy. The crucial role microgrids play in creating a dynamic, resilient energy environment is highlighted by the fact that choosing amongst different configurations depends on particular demands.
The Role of Energy Storage in Ensuring a Stable and Resilient Energy Supply
The cornerstone for ensuring a reliable and flexible energy supply system is energy storage. Its capacity to accommodate the fluctuation and intermittency of renewable energy sources, maintaining stability and resilience, underlies its varied role. Energy storage balances supply and demand by storing extra energy during times of high generation and releasing it during times of low production, reducing volatility and system instability.
Energy storage devices are an essential lifeline in emergencies like grid outages or natural catastrophes. They make it possible to do black starts, accelerate grid restoration, and assist isolated microgrids in maintaining vital services. Capabilities for voltage and frequency control further improve grid stability by preventing interruptions.
Peak shaving is another benefit of energy storage since it eases the burden during times of high demand and lessens reliance on expensive peak power sources. It gives islanded microgrids the ability to maintain a consistent energy supply, enhancing their resistance to outside disturbances.
Energy storage promotes a stable energy landscape by acting as a buffer against unpredictability. Its adaptability in emergency response, load control, and grid stability highlights how crucial it is to create a reliable and flexible energy future.
Solar Microgrid Implementation Challenges
As a sustainable answer to decentralized energy generation and delivery, the use of solar microgrids has drawn a lot of interest. To provide electricity to a particular area or community, a solar microgrid combines solar power generation, energy storage, and frequently additional renewable sources. Despite the obvious potential advantages, there are still several technical, governmental, and financial difficulties associated with the deployment of solar microgrids.
Addressing Technical, Regulatory, and Financial Challenges in Deploying Microgrids
Challenge | Approach to Address |
---|---|
Technical Challenges | |
Intermittency and Variability | Implement efficient energy storage systems (batteries) to store excess energy for use during periods of low solar generation. |
System Integration | Ensure compatibility of components through thorough system design and testing. Use standardized communication protocols for seamless integration. |
Voltage and Frequency Control | Deploy smart control systems that can manage voltage and frequency fluctuations in real-time, ensuring grid stability. |
Regulatory Challenges | |
Grid Interconnection | Collaborate with utilities to develop clear interconnection standards and protocols. Demonstrate the benefits of microgrids in enhancing grid resilience and reliability. |
Licensing and Permitting | Advocate for streamlined permitting processes specific to microgrid installations. Work with regulatory authorities to establish clear guidelines for microgrid development. |
Tariffs and Compensation | Engage with regulatory bodies to establish fair compensation mechanisms for energy sold back to the grid. Promote net metering policies to encourage microgrid adoption. |
Financial Challenges | |
High Initial Costs | Seek funding through grants, subsidies, private investments, and community partnerships. Explore innovative financing models like power purchase agreements (PPAs) to lower upfront costs. |
Return on Investment (ROI) | Develop robust financial models that consider factors such as energy prices, maintenance costs, and equipment lifespan. Use conservative estimates to demonstrate potential ROI. |
Lack of Financial Models | Collaborate with financial institutions and energy experts to create standardized financial models for evaluating microgrid projects. Share case studies to provide real-world data for modeling. |
Case Studies of Successful Microgrid Projects and Lessons Learned
- Brooklyn Microgrid, New York, USA
A peer-to-peer energy trading network called the Brooklyn Microgrid enables locals to purchase and sell energy produced by rooftop solar panels. Lessons learned include the significance of community involvement, regulatory innovation, and the necessity of participants having clear contractual frameworks.
2. Â Loiyangalani Solar Microgrid, Kenya
Situated in a rural area, the Loiyangalani Solar Microgrid offers dependable and clean electricity to a community that was not previously served. This initiative made it clear how crucial it is to combine solar energy with energy storage to guarantee a constant supply of electricity, as well as how important it is to form partnerships between the public and private sectors to overcome financial obstacles.
3. Â Sonoma Clean Power Microgrid, California, United States
During grid failures, the Sonoma Clean Power Microgrid is intended to offer resilience. Through this effort, it was made clear how important it is for local governments, utilities, and technology companies to work together to overcome tricky regulatory landscapes and gain funding for resilient infrastructure.
Implementing solar microgrids has many advantages but also presents several difficulties. A multifaceted strategy involving technical innovation, legislative change, and innovative finance structures is needed to address these issues. Stakeholders may promote sustainable and resilient energy systems through solar microgrids by studying successful case studies and putting their lessons into practice.
The Future of Solar Microgrids
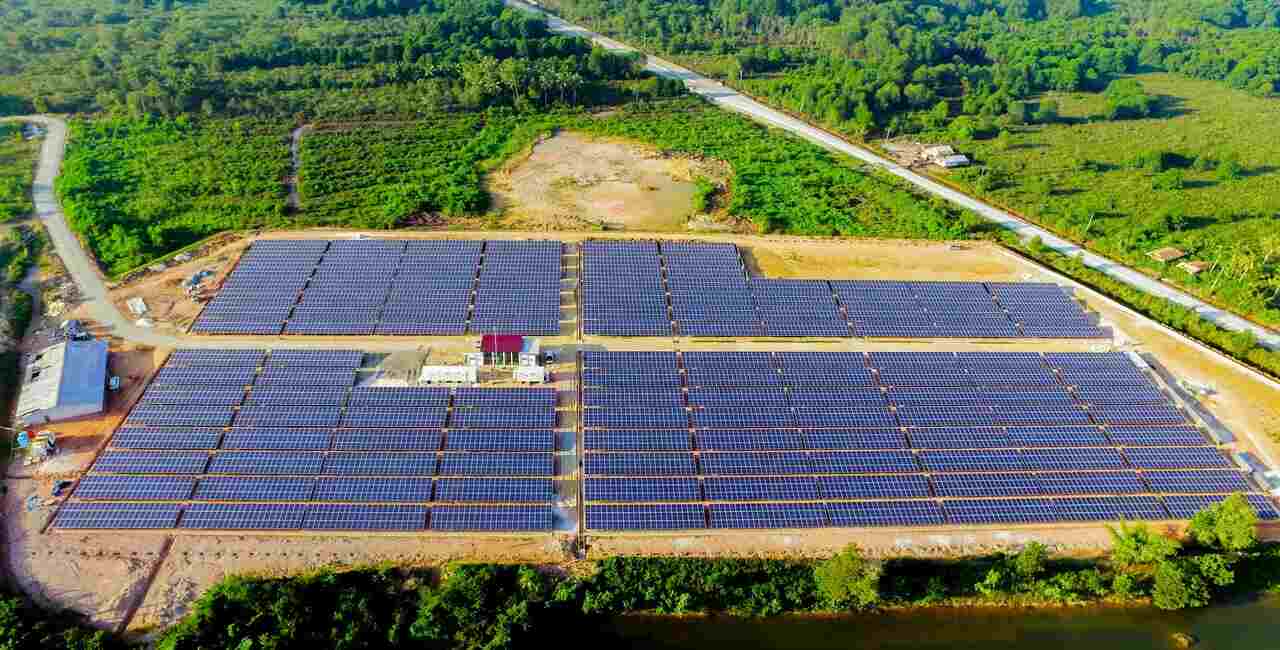
Innovative solutions are developing to redefine the way we produce, distribute, and use energy as the globe struggles to address urgent energy concerns including climate change, the depletion of fossil fuel supplies, and the need for reliable and resilient power infrastructure. Solar microgrids have emerged as one of these alternatives that have promise for changing the energy environment.
These localized, decentralized energy systems are gaining popularity because they have the potential to provide an effective and sustainable solution to the world's energy problems.
Exploring the Potential of Solar Microgrids
By integrating modern control and management systems with renewable energy sources, notably solar electricity, solar microgrids provide a novel method for distributing energy. Communities, business complexes, universities, and even remote locations may produce and manage their electricity with the help of these microgrids, which can work independently or in cooperation with the main grid.
The ability of solar microgrids to relieve pressure on centralized power generating systems, reduce transmission losses, and improve overall energy security is one of its most important benefits.
Additionally, solar microgrids are essential in the switch to greener energy sources since they lessen dependency on fossil fuels and greenhouse gas emissions. Increased energy resilience in the face of natural catastrophes, grid outages, and other disturbances results from giving communities the capacity to take control of their energy production.
Solar microgrids support local energy production, which promotes the development of sustainable energy ecosystems as well as the creation of jobs and economic growth.
The Role of Smart Technologies and Advancements in Enhancing Microgrid Efficiency and Scalability
Unlocking the full potential of solar microgrids depends on the development of smart technologies and innovations. The Internet of Things (IoT) and artificial intelligence (AI) are being effortlessly incorporated into microgrid operations as they continue to develop, increasing productivity and scalability. Within microgrids, smart technologies allow for the real-time monitoring, optimization, and control of energy production, consumption, and storage.
Artificial intelligence-driven algorithms are capable of predicting trends in energy consumption, allocating solar resources optimally, and effectively managing energy flows. AI can use data from a variety of sources, such as weather predictions, load profiles, and equipment performance, to make wise decisions that guarantee the highest level of energy efficiency and cost savings. Additionally, AI-driven predictive maintenance can increase the lifespan of microgrid parts and avoid unplanned outages.
Advanced batteries and other energy storage technologies are also essential parts of solar microgrids. These batteries make it possible to store extra solar energy during bright times and release it on gloomy days or times of high demand, assuring a steady supply of electricity. Smart controls and energy storage integration enhance the stability and dependability of the grid.
The general adoption of solar microgrids depends in large part on their capacity to scale. Solar microgrids are becoming more widely available to a wider range of consumers, from rural villages to metropolitan centers and huge industrial complexes, as technology advances and costs fall. The deployment of microgrid systems on various scales is made possible by standardized parts, modular designs, and expedited installation procedures.
Future solar microgrid development is anticipated to accelerate. To improve the effectiveness, dependability, and integration of these systems, governments, businesses, and academic institutions are investing in research and development. Collaboration among many stakeholders is essential for establishing beneficial policy frameworks, encouraging investments, and promoting innovation in the industry.
Conclusion
The establishment of solar microgrids offers a game-changing method for creating reliable off-grid energy systems. These microgrids make use of renewable solar energy to provide communities and businesses with a sustainable power supply as well as increased energy independence and dependability. Such systems require careful planning, the use of cutting-edge technologies, and community involvement in their design and execution.
Solar microgrids are emerging as a leader in innovation as the globe works to reduce climate change and guarantee resilient electricity access. They promise to empower rural communities, improve disaster preparation, and promote a more sustainable energy future.